This
page was produced as an assignment for an undergraduate course at Davidson
College.
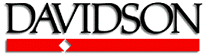
Telomerase
A SOLUTION TO THE "END-REPLICATION" PROBLEM
(click here for a review of DNA
replication)
- Because
DNA polymerases operate only in the 5' to 3' direction, synthesis of the
lagging strand occurs through a "backstitching" mechanism that produces
short fragments of DNA. This technique causes a problem at the end
of a linear chromosome: there isn't enough room for the RNA primer needed
to start the last fragment at the chromosome's 3' end. Without its
complement, the hanging piece of unpaired DNA from the parent strand might
be recognized by the cell as a broken piece of DNA and then damaged or
even chopped off in the cell's attempt to mend it.
- To prevent
the "correction" of the unpaired but important piece of DNA, eukaryotes
use certain G-rich nucleotide sequences as protective "caps."
Called telomeres, these
caps incorporate many tandem repeats of the nucleotide sequences (human
telomeres extend for about 10,000 nucleotides), ultimately allowing
efficient replication of the chromosome ends (Alberts et al, 2002).
- Telomeres
are created by telomerase, a
riboprotein complex that uses its own piece of RNA primer to elongate the
chromosome ends until the DNA polymerase can move in and complete the
lagging strand.
WHAT DOES TELOMERASE DO?
- When a
chromosome is replicated, the 3' hanging parental fragment containing the
G-rich sequence GGGTTA (in humans) is recognized by the telomerase
complex. Using its own RNA as a complemantary primer, the telomerase
mimics reverase transcriptases and elongates the hanging DNA fragment in
the 5' to 3' direction, moving down the growing strand until it is a
telomere several thousand bases longer. Replication of the lagging
strand can now be completed using the telomeric extensions as a template
for synthesis using DNA polymerase (Alberts et al, 2002).
- With this
mechanism, of course, the 3' end of each telomere is still slightly longer
than the complementary 5' end. Other telomere-associated proteins
move in to loop the protruding end back on itself, protecting it from
degrading enzymes and other mechanisms for repairing broken DNA.
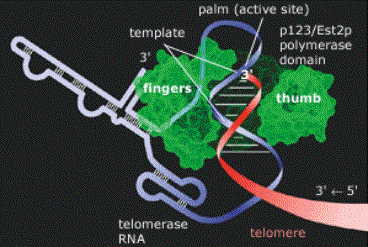
Figure 1: Telomerase Structure at Work. The
telomerase enzyme complex consists of an RNA component and a protein component.
The protein component's catalytic subunit acts as a reverse transcriptase
(green), using telomerase RNA (blue/white) as a template for the addition of
telomeric repeat sequences to the telomere DNA strand (red).
Source: Thomas Cech
TELOMERASE STRUCTURE AND HOMOLOGS
- TERT, the gene
for the telomerase catalytic subunit (the reverse trascriptase mimic) is
approximately 4 kb in length and exists somewhere in the chromosome as a
single-copy gene (Kilian et al,
1997). The catalytic subunit, named TP2, has a molecular weight of
approximately 130 kDa. Shaped almost like a hand as seen in Figure
1, its "fingers" and "thumb" pull the telomerase
RNA and telomere DNA strands into the active site (the "palm"),
where the telomere is elongated. The primary structure (amino acid
sequence) of TP2 is shown in Figure 2a (Harrington et
al, December 1997).
- The other major
telomerase protein is the RNA-binding subunit, named TP1; its amino acid
sequence is shown in Figure 3a. The amino-terminus of TP1 is
sufficient to bind telomerase RNA in vivo (Harrington
et al, January 1997).
- Both TP1 and
TP2 have similarities with telomerase-binding proteins of other species.
The amino-terminus of TP1 is both an RNA binding site and the
sequence segment most homologous to Tetrahymena p80, a ciliate
RNA-binding protein, as seen in Figure 3b, and Figure 2b
demonstrates the amino acid sequence similarity between the Est2 (yeast),
p123 (ciliate), and TP2 telomerase catalytic subunits. These results
suggest that the components of the telomerase complex are evolutionarily
conserved as homologous structures.
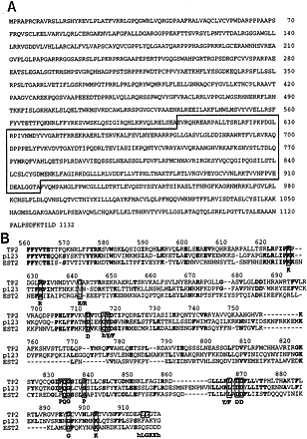
(click picture to see larger image)
Figure 2: Amino acid sequence of human TP2.
(a) Predicted amino acid sequence of human TP2. Homology to the
reverse transcriptase domain is boxed with a solid line. (b) Domain of
highest homology between TP2 (human telomerase catalytic subunit), p123
(ciliate Euplotes aediculatus ~123 kDa telomerase catalytic subunit),
and Est2 (yeast Saccharomyces cerevisiae homologous
telomerase-associated subunit). Residues conserved in at least two of the
aligned proteins are shown in boldface type. Residues conserved among
reverse transcriptase are boxed, with consensus residues shown below.
Source: Harrington
et al, December 1997
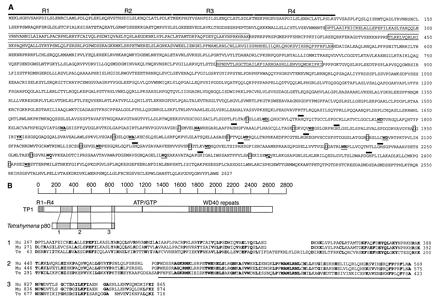
(click picture to see
larger image)
Figure 3: Amino Acid Sequence of Human TP1. (a)
Four amino-terminal repeats are indicated by solid lines over the sequence (R1
to R4). Boxed regions show homology to Tetrahymena p80. The
complete human and murine TP1 sequences have been deposited with GenBank
(accession numbers U86136 and U86137, respectively). (b) (Top)
The TP1 amino acid sequence is depicted schematically, with boxed regions as
follows: amino-terminal repeats 1 to 4 (R1-R4); gray boxes (1 to 3) show
regions of homology between TP1 and Tetrahymena p80. (Bottom)
Human (Hu) and murine (Mu) TP1 amino acid sequences were aligned to Tetrahymena
p80; Tetrahymena (Te) sequences identical to either human or mouse at
each position are shown in boldface. The underlined, 90-amino acid segment in
region 2 contains 46% identity between p80 and human TP1.
Source: Harrington
et al, January 1997
- In
addition to the telomerase protein components showing similarities, the
telomerase RNA itself shows signs of being at least partially conserved.
The TP1 and p80 similarities at the amino-termini suggest that at
least the telomerase-binding sites must be similar between the two
species' RNA strands. As Figure 4 shows, possible secondary
structures of the two species' RNA may be similar enough to be recognized
by an evolutionarily conserved RNA-binding motif.
a
b
Figure 4: A Comparison of Human and Yeast/Ciliate
Telomerase RNA. Vertebrate/human telomerase RNA (a) and yeast/ciliate
telomerase RNA (b) differ greatly in sequence and structure, but they share a
5' pseudoknot area close to the template sequence (i.e. the portion used to
generate the telomere).
Source: (a) Rfam
3.0 Telomerase-vert
(b) Rfam 3.0
Telomerase-cil
LOCATING TELOMERASE IN THE GRAND SCHEME OF THINGS
-
Telomerase is found in the nucleus, where DNA replication takes place.
Not all cells, however, show evidence of telomerase activity.
Expression of TERC, the gene for the RNA component of telomerase, is
widespread, but the expression of the protein component's catalytic
subunit, TERT, is restricted to locations of telomerase activity.
Telomerase activity was found to be almost absent in the majority of
normal adult tissues, including cardiac and skeletal muscle, adipose
tissue, lung, liver, and kidney (Kolquist
et al, 1998).
- Because
of this curious lack of telomerase activity, a theory arose connecting
telomere length to aging and cell senescence. According to this
theory, human somatic cells are born with a full number of telomeric
repeats, but the telomerase enzyme is "turned off" in some
tissues. The cells of those tissues would lose about 50 to 100
nucleotides from each chromosome end each time they underwent replication
and division. Eventually, the telomeres would cease to exist and the
chromosomes themselves would start losing nucleotides, carrying genetic
defects into their next division so that neither daughter cell would be
viable and would consequently undergo senescence (Alberts et al, 2002).
Thus after a certain number of divisions a cell has "aged"
and died.
- This
theory has been tested using human fibroblasts grown in tissue culture.
Human fibroblasts normally divide around 60 times before dying, and
like most other somatic cells, they do not produce telomerase. Their
telomeres gradually shorten as they continue dividing. When the
telomerase gene is added to the cells' genomes, however, they maintain
their telomere length, and some of the cells are able to proliferate indefinitely.
- If
telomere length is a cause of human aging, then, might exposure to
telomerase lengthen one's life?
- A control
on cell division certainly seems important, however, in light of the fact
that approximately 85% of all tumors show evidence of telomerase activity
(Kilian
et al, 1997). If telomerase is provided to maintain telomere
length, a cell's life may be prolonged indefinitely, causing genetic
instabilities that in turn lead to variant, cancerous cells. Figure
5 compares telomerase gene expression in normal human tissue and
concerous tissue, suggesting the correlation.
`
Figure 5: TERT Expression in Colonic Tumorigenesis.
(a) Normal colon with low-level hybridization to epithelial cells
located primarily in the proliferative zone near the base of crypts. (b)
Increased expression in adenomatous epithelium (right), normal mucosa is on the
left. (c) Increased expression in carcinoma in situ (bottom) arising in
an adenomatous polyp. (d) Strong expression in colon carcinoma metastatic
to liver.
Source: Kolquist
et al
TELOMERASE MUTANTS?
- Telomerase has
often been mutated in lab settings for studies on its structure and
activity, but I have not come across mention of a "natural"
mutation.
- A widely-publicized
mutation was created in 2001, when UCSF scientists created a tiny mutation
in the RNA template that caused the telomeres to be unstable after a few
divisions. The mutation caused a dramatic halt in cancer cells'
replication and division, in effect telling the cancer cells to commit
suicide. As a potential "cure for cancer," it's still
being researched in the hope that it will yield a treatment that doesn't
damage normal human tissue the way other cancer treatments do (Trinkl,
2001).
REFERENCES
- Alberts, et al. Molecular
Biology of the Cell. New York: Garland Science, 2002.
- Kilian, et al.
Isolation of a candidate human telomerase catalytic subunit gene,
which reveals complex splicing patterns in different cell types. Human
Molecular Genetics; 1997 (12), 2011-9. <http://hmg.oupjournals.org/cgi/content/full/6/12/2011>
Accessed 2003 March 12.
Molecular
Biology Homepage
Sarah's
Molecular Homepage
Email Sarah